"Truth is stranger than fiction; fiction has to make sense."
- Leo Rosten
Quantum Mechanics: An Introduction
Part 1 of "An Introduction to Quantum Reality"
At the smallest, subatomic level, quantum mechanics has made remarkable discoveries about the behaviour of elementary particles. It would seem logical to assume that by studying these fundamental building blocks of our universe we might gain some of our best insights into the true nature of reality.
The predictions of quantum mechanics are very much driven by experimental results. Quantum mechanics does not have much to say about "why" things happen, it can just be used to predict "how" things behave, that behaviour being based on the well-established results of experiments. And the most famous of those experiments is the double-slit experiment.
In September 2002, the double-slit experiment was voted "the most beautiful experiment" by readers of Physics World (see here). Richard Feynman is said to have remarked that it contains everything you need to know about quantum mechanics. But perhaps the most useful property of the experiment is that it shows just how weird quantum reality can be!
In the double-slit experiment an electron gun is aimed at a screen with two slits, and the positions of the electrons is recorded after they pass through one of the two slits, making little dots on the screen. It is found that an interference pattern is produced on the screen just like the one produced by diffraction of a light or water wave passing through the two slits. There are bright bands ("constructive interference") and dark bands ("destructive interference").
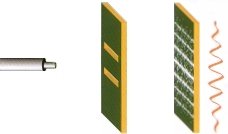
This is quite strange: electron particles are interfering with each other as if they were waves. However, things turn much more weird if we only emit one electron at a time. We wait until an electron makes a dot on the screen before emitting another electron, so there is only ever one electron in the system at a time. A pattern slowly builds on the screen, dot-by-dot, as each individual electron hits the screen. What we see is quite incredible: the accumulation of dots on the screen eventually produces a pattern of light and dark bands - an interference pattern emerges even though there is only one electron in the system at any one time! The electron appears to be interfering with itself. So in some strange way it is as if the electron goes through both slits at once!!
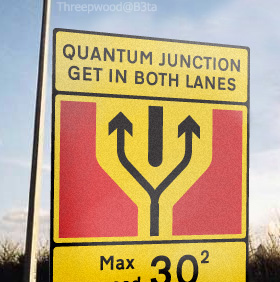
How can this be? Maybe half the electron is going through one slit and half through the other slit? But if we put a small detector screen on the other side of one slit we only detect whole electrons sometimes passing through (sometimes passing through the other slit). It's as if the electron does indeed pass through both slits at once, but when we make an attempt to detect it, it suddenly decides to act like a single particle which has gone through just one slit!
It is only in the last ten years or so that quantum mechanics has at last been able to shed some light on what is happening in the double-slit experiment (this will be considered later in the page on Quantum Decoherence), but before we get to that stage there is a fair bit of theory to be covered. And it's best to start at the beginning.
Quantum mechanics could be said to have started in 1900 when Max Planck made the discovery that light, which was considered to be purely wave-like, was in fact composed of energy which came in discrete packets (called "quanta"). In the Planck formula, the energy of the packets, e, is proportional to the light frequency, f, the constant of proportionality being Planck's constant, h:

This result suggested that waves (light) were in fact composed of particles.
The converse of this result came in 1923 when Louis de Broglie (pronounced to
rhyme with "destroy") suggested that matter (particles) behaves as a wave (as is
evident in the double-slit experiment), the wavelength, , being
inversely proportional to the particle's momentum, p. Here's the
derivation:
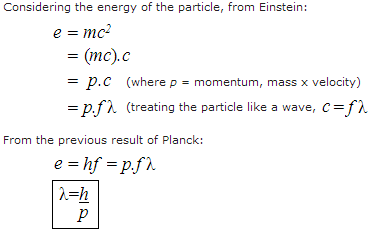
We now know that absolutely everything in the known universe is made out of these strange particle/wave entities which obey these two formulae for quantum behaviour, given above.
How can we make sense of this strange wave/particle duality? Would it be possible to combine these two results in a single equation, and in the process reveal more insights into the true nature of reality at the quantum level?
Yes, Erwin Schrödinger did it in 1926. And we'll see that in the next page on The Quantum Casino.
The Heisenberg Uncertainty Principle
If we have a wave (say, a light wave) which has a corresponding particle
representation (in this case, a photon particle), we can model what happens when
we try to work out precisely where the particle is (in this case this would
happen when we shine a light wave on a screen, for example, and detect the
photon's position). We can model what happens when we try to localise the
position of the particle in this manner by adding a series of waves with similar
frequencies (for an interactive example, go here
and add three waves with amplitude of 20 and wave numbers 0.22, 0.23 and 0.24).
The resultant wave will have a narrower spatial extent and is called a
wavepacket. The width of the wavepacket is determined by the difference
in the wavelengths of the original wave and the modulating wave: .
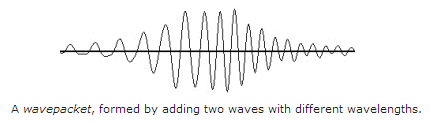
The wavepacket idea can be used to localise the wave (i.e., make it a
localised particle) in a narrow extent (which
will be equal to
).
The resultant uncertainty (spread) in position of
the particle, , multiplied
by the uncertainty (spread) in the momentum of the particle,
,
is then given by:
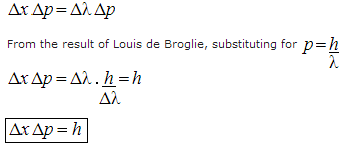
which is the Heisenberg Uncertainty Principle (for more details, see here).
The Heisenberg Uncertainty Principle tells us that the more accurately we
determine a particle's position, , the less
accurately we can know its momentum,
. This Uncertainty Principle equation has been used to generate the
animation below:
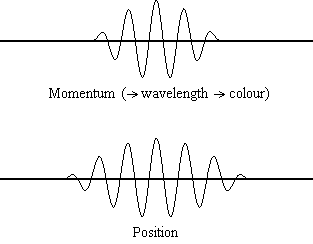
The animation shows the relevant spreads in the uncertainty for position and
momentum (for a light wave, and the light wave's corresponding photon particle).
From the result of de Broglie (considered above) we know that if we have a
precise value for a particle's momentum, p, then we have a precise
value for the wavelength of the wave, , and
hence a precise colour of our light wave. A precise value for particle momentum
is shown in the above animation when the momentum value becomes a single
vertical point. You will see that when that occurs, the corresponding
uncertainty in the particle's position spreads out to infinity, i.e., when we
have light behaving as a normal light wave (with a colour) we can have no idea
where the corresponding particle is. In the wave/particle duality model this
shows the case when the light is acting like a wave, not a particle.
Conversely, the situation when we have a precise position for the particle, e.g., when we shine the light wave onto a screen and detect the proton, is shown in the above animation when the position value becomes a single vertical point. You will see that occurs, the corresponding uncertainty in the particle's momentum spreads out to infinity, i.e., we can now no longer determine the wavelength or the colour of the light wave. In the wave/particle duality model this shows the case when the light is acting like a particle, not a wave.
Comments
Here you can add your comments about this
article.
Your comments will appear instantly on this page (underneath this
form):
Hi Andrew, thanks for these wonderfully entertaining pages - I'm in the process of reading them all. I noticed a small typo on this page: s/proton/photon/g (1 occurrence). - John, 31st July 2007
Thanks so much for calling these pages "entertaining". That's probably the most satisfying thing you could have said. If I've succeeded in presenting physics in an entertaining manner (rather than dry and dull technical papers) then I'm very happy. Thanks also for the typo spot. I've fixed the text now. Typo and broken link reports are always very welcome. - Andrew Thomas, 31st July 2007
I'm no maths/physics student but surely if you measure a particles position TWICE you can determine it's momentum. If particle z is at location a,b,c (3d co-ordinates) and then measured one tick later at a2,b2,c2 why isn't it's momentum obvious? - Neil Barnes, 11th September 2007
That's a really good question,
Neil, but you're thinking in terms of classical physics. What you suggest is
perfectly correct for large, "ordinary objects" which are large enough so that
quantum effects are not evident. But down at the smallest scales, things just
don't work like that. When you start dealing with the smallest particles, they
behave very differently, counter-intuitively. After all, how would we measure
the position of the smallest, elementary particle? We could only do that by
interacting with it, by getting the original particle to collide with another
(measuring) particle - thus inevitably modifying the very position measurement
we were trying to obtain! Even with large objects we have to modify them when we
take a measurement. For example, when we take the temperature of an object we
have to remove a small sample of heat from the object. Or when you check the air
pressure in your car tyres you have to take a bit of air out of the tyres. These
effects are so small with large objects that we do not notice them (so your
proposal would be fine in that case), but we can't ignore these effects when we
deal with quantum objects.
So these elementary particles behave very
differently to "ordinary objects". They behave according to quantum mechanical
rules: sometimes they behave like a wave, sometimes like a particle. The more we
constrain one property (say, the position property) to act like a particle,
localised in space, the more the other property (momentum) acts like a wave,
spread out in space.
Wikipedia provides a helpful analogy with sound
waves which resembles your "two points in space" idea: "It is meaningless to ask
about the frequency spectrum at a single moment in time, because the measure of
frequency is the measure of a repetition recurring over a period of time.
Indeed, in order for a signal to have a relatively well-defined frequency, it
must persist for a long period of time, and conversely, a signal that occurs at
a relatively well-defined moment in time (i.e., of short duration) will
necessarily encompass a broad frequency band." See http://en.wikipedia.org/wiki/Uncertainty_principle
So the more you try to pin-down the frequency of a wave, you need to stretch out
your time measurements (the two position measurements you mention), and the more
accurately you know the time, the less accurate is the frequency measurement.
But thanks for your idea. You'd be correct for everyday objects, just
not for quantum mechanical objects. - Andrew
Thomas, 11th September 2007
In the Double slit experiment-If we shoot only one electron at a time at the two slitted screen, and do not attempt to measure which slit it went thru, do we get a separate interference pattern from each such single electron, or only from the conglomeration of many electrons.? - Paul Gayet, 3rd October 2007
I see what you're getting at, and the text was not clear about this. Each electron, after it has passed through the slits, makes only a single point on our detector screen: when we try to detect a particle, we see a particle. We see the interference pattern in the distribution of dots on the screen - that's when you see the light and dark areas. So we need many electrons making many dots. I've just amended the text to make that clearer. - Andrew Thomas, 3rd October 2007
Hi Andrew! I'm just a
dilettante reader about quantum physics, but even so I want to thank you for
stopping the final meltdown of all my neurons with your intelligible
explanations.
I'm just curious: there is no info about who you are and what
is your work and why you do this kind of mathematical charity ?!
All
comments date from few months ago. Hv u started this site so recently?
Hugs
Francisco Peres, 48, Curitiba - Brasil
francperes@uol.com.br
- Francisco
Peres, 4th November 2007
Hi Francisco, thanks for your comment. I've been doing this site for three years but it only went live this year. I do it because I love it and I'm fascinated by these subjects. The underlying principles are usually surprisingly simple, and I think it's important to get that across. - Andrew Thomas, 4th November 2007
what the double slit experiment is telling us is that subatomic particles are able divide their energy and still maintain the properties of the original particle. What enables them to rejoin? - eleeo, 13th November 2007
Eleeo, you'll have to read the remaining pages! Interpretations of quantum mechanics suggest that there is no such object as the "original particle" to which you refer. Instead, before we detect the particle, we must consider it to be unreal - and we have to consider it as being potentially in all positions at once! Only after we detect the particle does it become "real", with a clearly-defined position. But that's all covered in the later pages. - Andrew Thomas, 13th November 2007